Find out how you can capture 3D images with nanoscale precision, without scanning your z stage
Written by Karen McGeachy in conjunction with our partners Double Helix Optics. This blog is aimed to give you an overview of our SPINDLE® and SPINDLE² modules and show how Double Helix Optics’ Light Engineering™ technology can aid you in your imaging applications.
We will cover the following topics:
- Background on Point Spread Functions
- This section details the principle of Point Spread Functions, of which the Double Helix Optics Light Engineering technology is based.
- How does the Double Helix Optics Light Technology™ technology work?
- This section provides an overview of how the technology works and the benefits of it
- The SPINDLE Product Family – key benefits
- This section explores the two SPINDLE modules and outlines the benefits such as multichannel imaging on a single camera, simultaneous mulit-colour imaging, multi modal, and 3D and non 3D comparisons
- Software
- This section provides an overview of the software and its capabilities
- Applications
- This section goes into details of the multiple applications the technology has been used for such as 3D Single Molecule Localisation Microscopy, Particle tracking, Light sheet microscopy, Multiphoton, and beyond life sciences.
Written by Karen McGeachy
10-15 minutes Read

1. Introduction
Due to the diffraction of light, traditional light microscopy is unable to resolve structural details less than ~200 nanometres in the lateral dimension and ~500 nm in the axial dimension. For over a century, this was considered a fundamental, unbreakable rule.
After decades of gradual improvements, revolutionary change has arrived with the advent of superresolution imaging. Now it’s possible to see molecular structures as small as 10-20 nm in two dimensions. But this technology lacks axial resolution, failing to capture depth information.
With Double Helix Optics’ Light Engineering technology, it is now possible to capture 3D images with nanoscale precision. By modifying the point spread function (PSF) of the optical system, users of the SPINDLE® and SPINDLE2 imaging modules are able to capture details as small as 10-15 nm in the lateral dimension and 15-25 nm in the axial dimension. This has sweeping implications for advancing scientific research, drug discovery, and industrial inspection.
2. Background on Point Spread Functions
PSF, as the name suggests, is the response of an imaging system to a point source. This function describes the ‘spread’ due to the nature of the optical system. The final image is the convolution of the object with the PSF [Fig 1].
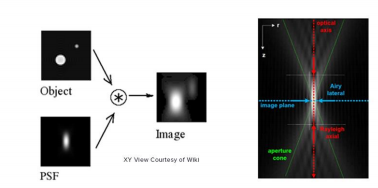
An optical system with resolution performance at the instrument’s theoretical limit is said to be diffraction-limited or Abbe Limit, which is proportional to the wavelength of light and the Numerical Aperture (NA). The NA can be thought of as the ability of a lens to collect light at oblique angles (n sinθ) and can be as high as 1.4 – 1.6. A nice rule of thumb is to half the wavelength, so around 200nm for visible light.
Resolution in microscopy has many different definitions but John William Strutt, 3rd Baron Rayleigh expanded on the work of George Airy and invented the ‘Rayleigh Criterion’ in 1896, which defines the diffracted limit as when the two point sources are distinguishable from each other.
As mentioned in the introduction, super resolution techniques (such as single molecule localisation microscopy) enable users to beat this criteria by temporally separating the two peaks and reporting on the peaks location, where the localisation precision is dependent on the photon flux.
3. How does the Double Helix Optics’ Light Engineering™ technology work?
Double Helix Optic’s Light Engineering technology alters the response system of the PSF of a microscope to capture information that is out of focus with the standard Airy disk of the typical microscope. The image below [Fig.2] demonstrates the ability of the double helix PSF to stay in focus above and below the focal plane while the standard Airy disk and astigmatic PSF go out of focus.
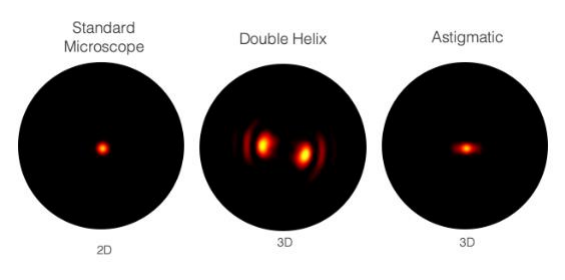
In conjunction with the SPINDLE or SPINDLE² modules, a phase mask inserted into the pupil plane modifies the PSF from the Airy disk of the microscope. With a library of e-PSFs available, performance is optimised by selection of the most appropriate phase mask.
The graphic below creates two lobes which rotate round a point, the location of the emitter is based on the centre point of the lobes and the axial position is based on the angle the two lobes make [Fig 3].
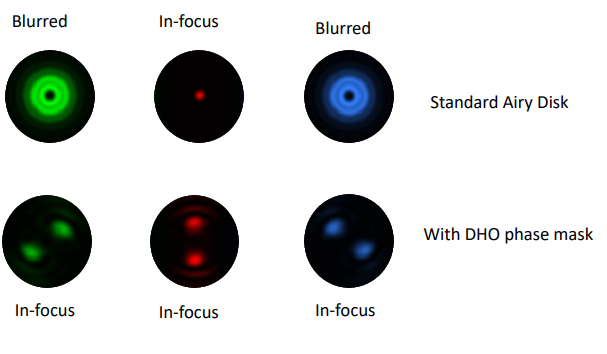
Depending on the objective in use, the double helix PSF offers up to 6 times the depth of a conventional microscope system. Additional masks offer up to 30x depth of a conventional microscope. This depth is achieved without the need for scanning the Z stage.
4. The SPINDLE® Product Family – Key Benefits
Double Helix Optics’ award-winning SPINDLE family enables easy capture, analysis and tracking of multicolour 3D images down to the single molecule and single particle level as well as extended depth whole cell imaging.
Designed as modular upgrades compatible with most scientific microscopes, the SPINDLE product family seamlessly extend the capabilities to enable 3D superresolution and extended depth of field imaging and tracking.
The SPINDLE and SPINDLE2 modules can be added at the camera port of Sa microscope [Fig 4].
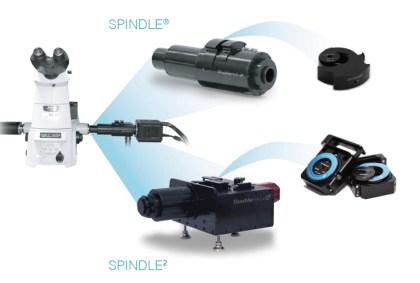
Science is an ongoing quest to bring new insight. To date scientists using optical microscopy have been limited in their ability to see structures in 3D and with precision below the diffraction limit.
With the SPINDLE and SPINDLE2 modules researchers now have the ability to easily capture and analyse 3D images of cellular structures and intra and inter cellular activity down to the single molecule level.
Optimised to ensure the best performance from the library of phase masks to engineer the PSF [Fig 5], the SPINDLE and SPINDLE² add 3D capabilities to your system matched to the precision -depth requirements of your experiment.
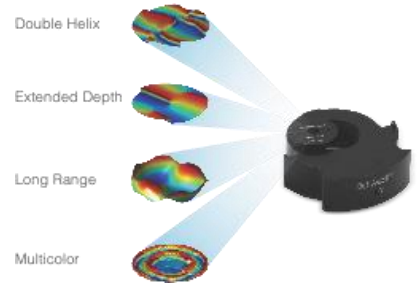
Features of the SPINDLE2 – Multichannel imaging on a single camera
Like the SPINDLE, the SPINDLE² attaches between your microscope and camera. Light from the microscope is split into two paths [Fig 6] by:
- Emission wavelength (using a dichroic beamsplitter)
- Intensity (using a standard beamsplitter),
- Polarisation (using a polarising beamsplitter)
and then combined back onto the camera as side by side images.
Each channel can be PSF engineered or left in bypass mode. There are several advantages of imaging on a single camera as opposed to two cameras, an important one being the cost factor.
However, there is also the consideration that there is no need for synchronization between the cameras which can often be complex.
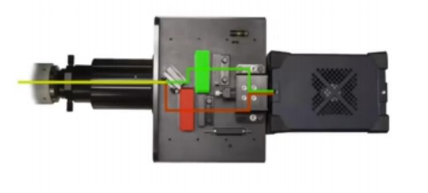
Simultaneous multi-colour imaging
The SPINDLE² allows for simultaneous multicolour imaging for simultaneous study of two tagged species. Utilizing your phase masks of choice for each channel you can track and analyze colocalization, utilize one channel for fiducial and the second channel for imaging, and track fiducials to merge multiple captures to extend depth range even further. With the multi-colour phase mask option, you can image up to 4 colours simultaneously.
Depending on the phase mask of choice users can realize increased depth range of up to 30x clear aperture, without the need to scan the Z stage. This capture of more data in a single image not only saves significant time performing experiments but also reduces phototoxicity of sample.
Multi modal
With the SPINDLE2 users can chose between multiple imaging modalities including 3D particle tracking, 3D Single Molecule Localization Microscopy (SMLM), FRET, SOFI, widefield and more. For example, you can use chose to use a phase mask in one channel and polariser or even a second focus point lens in the second channel.
3D and non 3D comparisons
Another advantage of the SPINDLE² is the ability to easily switch between two channels, single channel, multi-focus or by-pass mode for non-3D experiments. This is particular useful if the user would like to compare between 2D and 3D simultaneously.
5. Software
The data obtained using the SPINDLE® and SPINDLE² can be analysed using Double Helix Optics’ 3DTRAX® software [Fig 7] designed as an easy to use ImageJ/FIJI plugin. You would capture the data using the same tools that you are familiar with on your microscope and then import them to 3DTRAX for processing and analysis.
Key features of the software:
- Modules available for 3D SMLM, 3D tracking and extended depth of field whole cell imaging.
- For 3D SMLM and 3D particle tracking, the x,y and z position of every particle is calculated, utilizing proprietary algorithms to automate 3D localization.
- Renders highly precise 3D images (<30nm) with unprecedented depth and resolution for single molecule localization and tracking.
- Automates drift correction and provides intuitive plots to preserve data quality.
- Intuitive plots help ensure quality data throughout the analysis process.
- Allows users to save images in ThunderSTORM or Double Helix file formats and export files for further analysis.
- Extended depth of field reconstruction allows imagining of whole cell, in a single capture, without depth scanning.
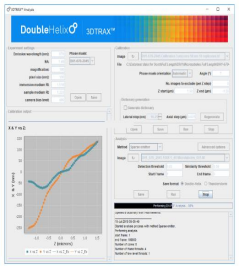
6. Applications
3D Single Molecule Localisation Microscopy
Single Molecule Localisation Microscopy (SMLM) are a family of techniques, including STORM, PALM and DNA PAINT, which offer the highest resolution methods.
This family of methods tags biological samples by attaching synthetic dyes to target molecules. These dyes are able to enter a blinking state so that only a small number of them are “on” during an image frame.
By collecting many frames, often thousands, you can reliably sample the underlying structure and identify the position of the single-molecule to a sub-diffraction localisation.
Adding the Double Helix Optics’ SPINDLE® enables the recovery of up to 10x more depth information than in a 2D SMLM experiment.
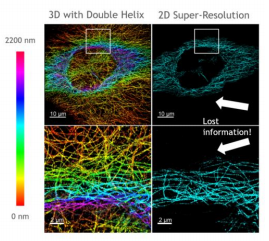
In [Fig 8], you can see sharp, colour-encoded depth throughout the sample in contrast to 2D imaging, where much of this information is out of focus and lost, hiding the structure of the sample.
RHS shows 2D super-resolution image and LHS shows the same sample but with the SPINDLE® for 3D imaging.
SPINDLE² expands on this by offering the same 3D information but with multi-colour capabilities [Fig 9].
The 3D nature of the reconstruction is particularly beneficial for these complex three dimensional networks.
Fig 8 & 9 – Images courtesy of Double Helix Optics.
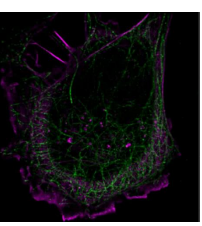
Particle tracking
Conventional 2D tracking requires axial stitching to extend the imaging volume and not lose particles to defocus. Utilising the SPINDLE® and SPINDLE² in conjunction with Double Helix Optics’ 3DTRAX software, as the particle moves in the axial position the data is recorded in the angle that the lobes make. So now we can track these particles in 3D with remarkably high precision, enabling a much better reconstruction of the particle’s overall trajectory [Fig 10].
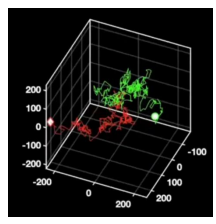
For a more in depth look at how the SPINDLE and SPINDLE² can be used for 3D particle tracking with examples such as liquidsolid surface diffusion, understanding cell signaling and diffusion and co-localisation, please see the Double Helix Optics Webinar – http://www.doublehelixoptics.com/2020/06/02/webinar-dholight-engineering-for-3d-single-molecule-tracking/
Light sheet – 3D single-molecule super-resolution microscopy with a tilted light sheet
Light Sheet microscopy is a growing field. Unlike traditional illumination methods a light sheet illuminates a thin section of the sample that is orthogonal to the detection path. The light sheet reduces background, increases signal-to-noise ratio and reduces photobleaching of the sample.
However conventional light sheet methods do not allow imaging with high-NA objectives close to the coverslip. In this application from the Moerner Lab at Stanford University, the team combined the powerful methods of light sheet and high-precision 3D SMLM, creating a technique known as “TILT3D”.
Combining light sheet with the Double Helix and Tetrapod PSFs they were able to image a complete HeLa cell with super-resolution precision.
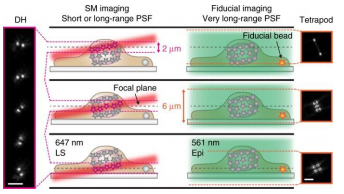
To do this they matched the thickness of the light sheet with the depth of focus of the Double Helix PSF. They then used a long-range Tetrapod PSF to track fiducials on the coverslip of the imaging chamber. By tracking the fiducials while imaging separate slices of the sample with the Double Helix PSF they were able to image and reconstruct structures throughout whole mammalian cells.
Gustavsson, A., Petrov, P.N., Lee, M.Y. et al. 3D single-molecule super-resolution microscopy with a tilted light sheet. Nat Commun 9, 123 (2018). https://doi.org/10.1038/s41467-017-02563-4
Multiphoton – Two-Photon PSF-engineering image scanning microscopy
This application from the Piestun Lab at the University of Colorado and used a two-photon fluorescence image scanning microscope (ISM) in conjunction with engineered excitation and detection PSFs enabling 3D imaging in a single 2D scan.
Excitation was from a holographic multisport array of focused femtosecond pulses and the Single Helix PSF. This enabled volumetric imaging of biological samples over a depth of field spanning more than 1500nm and with an axial resolution of better than 400nm
Omer Tzang, Dan Feldkhun, Anurag Agrawal, Alexander Jesacher, and Rafael Piestun, “Two-photon PSF engineered image scanning microscopy,” Opt. Lett. 44, 895-898 (2019)
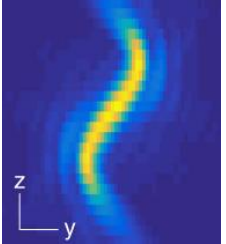
Beyond life sciences
Double Helix Optics Light Engineering technology extends beyond super-resolution microscopy and extended depth of field imaging for life sciences to applications including industrial inspection and machine vision. The principle of engineering the PSF can be applied to any imaging system by scaling the size of the phase mask to the imaging system. When applied to the field of machine vision for example, the approach offers several advantages over existing methods such as stereo-vision, structured illumination, time of flight, and light field:
- Incorporating a phase plate reshapes the focal point to enable depth capture with limited impact on 2D system performance and with minimal shadowing.
- The depth resolution and depth of field of the 3D imaging system can be optimised by designing the PSF to match the 2D lens in use.
- The engineered PSF technology can be implemented as an add-on to existing 2D imaging systems or as phase plates that can be incorporated into an existing 2D lens system.
- For 3D machine vision systems designed for engineered PSF, the phase plates add effectively zero to the volume or weight and no second camera or additional light sources are needed in most instances.
For more information on how the technology can be used in machine vision please see the following article – https://www.photonics.com/Articles/Optical_Advancements_Enable_HighPrecision_3D/a64430
For in depth look at applications see Double Helix Optics’ page which includes a list of publications and specific application notes – http://www.doublehelixoptics.com/applications/
7. Summary
Hopefully we have answered some of the questions asked by customers and shown the benefits of adding either single channel or dual channel 3D nanoscale imaging to your set up. Below is an overview of the key advantages of Double Helix Optics’ Light Engineering technology and what the SPINDLE and the SPINDLE² bring to your experiment.
Double Helix Optics’ Light Engineering technology
- Enabling extended depth imaging with unprecedented combination of precision – depth
- Depth from defocus
- Depth without scanning
- From nanometer to millimeter sized objects
The SPINDLE® family
- Up to 30x depth of conventional microscope in single image
- An affordable upgrade to existing microscope systems
- A range of imaging modalities – from 3D SMLM to whole cell to extended depth of field industrial inspection
- SAdaptable to customer’s experimental/imaging/tracking requirements
- Simplicity of use
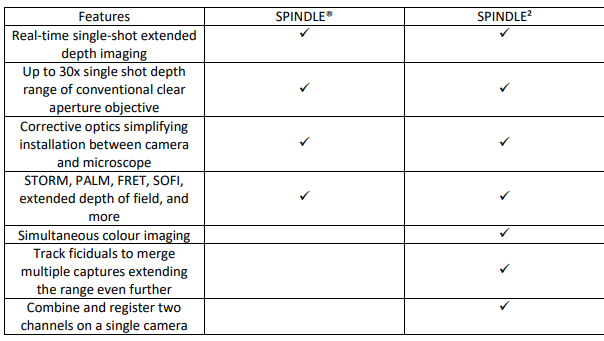
Want a loan unit to test in your application?
Contact us here:
Other Blogs/Articles that may be of interest:
- Tilted Light Sheet with DHO PSF Engineering for 3D Whole Cell and Sub-Cellular Super Resolution Imaging
- Understanding the jargon of LCOS Spatial Light Modulators (SLMs)
- Spatial Light Modulator Applications
- Fluorescence Microscopy Light Source: LED vs Bulbs
- Confocal Microscopy – The Re-Scan Confocal Microscope (RCM)
- What are the benefits of using a fixed Femtosecond Fibre Laser in Two-Photon microscopy